SOS from the mantle : the leaking roof
- Dohyeon Lee
- Jun 10, 2017
- 3 min read
“Mr. Mantle thought that he had only one problem with his house, the holes called ‘subduction zone.’ However, recently he heard the shocking news that he had a leaking roof too.......”
Serpentinization of upper mantle at subduction zones can be explained by dehydration of a subducting plate. But there has been no proven mechanism for serpentinites beneath slow-spreading mid-ocean ridges and most of rifted continental margins.
In March 2016 issue of Nature Geoscience, Bayrakci et al. showed that serpentinization at the rifted continental margin offshore from western Spain was probably initiated when the whole crust cooled to become brittle and deformation was focused along large normal faults. They also estimated the fluid flux along the faults and found it is comparable to that inferred for mid-ocean ridge hydrothermal systems. They concluded that brittle processes in the crust may ultimately control the global flux of sea water into the Earth(ref.1).
When the mantle peridotite reacts with hydrothermal fluid, it alters to serpentinite. At a magma-poor rifted continental margin, during rifting, crustal thinning leads to a reduction in overburden pressure at depth, which together with cooling moves the originally viscous lower crust into the brittle field(ref.2). It allows the fault to be extended to the boundary between the crust and mantle. The normal faults, which have the smallest dips, form a detachment-like surface between the fault-bounded tilted crustal blocks and the upper mantle. Serpentinization occurs along this boundary, and the serpentinized layer is described from seismic reflection profiles as the `S reflector'(ref.3). So if S reflector is found, it can be assumed that there were input of fluid through the crust, because the presence of S reflector implies that serpentinization had occurred.
The authors thought that the fluid would be supplied along faults, and they tried to find the evidence from the relationship between faults and serpentinization by using seismic tomography and numerical model that calculates the thickness of crust where the entire crust becomes brittle in North Atlantic rifted margins. They made 3D seismic tomography with higher resolution of the Deep Galicia margin, one of the forementioned margins. Then, they estimated the water supply to the mantle via faults from the volume of serpentinites and the degree of serpentinization.
The model results were compared with seismic tomography data. Serpentinites were found only where the entire crust is brittle and the normal faults on the crusts are block-bounded, which enables water to penetrate into the mantle. This implies a possibility that normal faults cutting down from the crust to the mantle would control the serpentinization process.
Figure 1 shows the seismic tomography data of the Deep Galicia margin. Below the S reflector, 6.5 and 7.5 km/s iso-velocity contours correspond to ~45% and ~15% serpentinization, respectively, if these velocity variations are attributed solely to variations in the degree of serpentinization(ref.4). Therefore, the larger the depth difference between the two contours, the higher the degree of serpentinization. The highly serpentinized zones are localized under the hanging walls of normal faults, which could lead to the interpretation that serpentinization was caused by sea water coming along the faults.
The net time-integrated downward flux for each fault is about tens of thousands ㎥ per square meter of fault cross-sectional area. This values are comparable to that of mid-ocean ridge faults in the Oman Ophiolite(ref.5).
In this paper, the authors assumed that S reflectorㅡthat is, serpentiniteㅡwould give information on the pathway of water transport at crust-mantle boundary. They provided geophysical evidences for the mechanism how water immigrates to the mantle through the faults at continental rifted margins and tried to quantify the amount for which this mechanism accounts in earth’s water cycle.
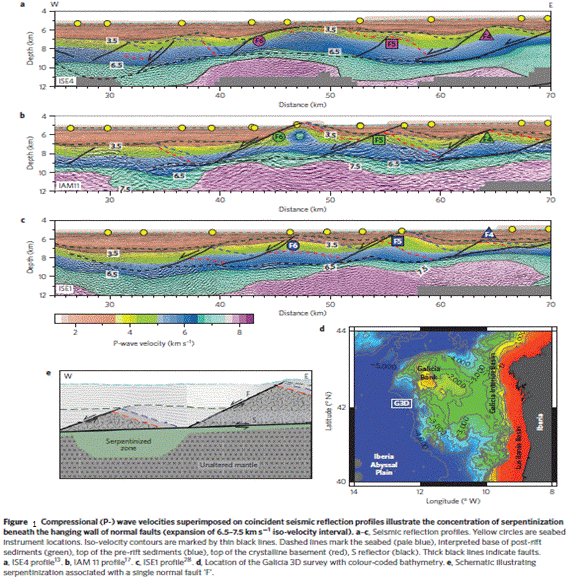
REFERENCES
1. G.Bayrakci et al., Fault-controlled hydration of the upper mantle during continental rifting, Nature Geoscience 9, pp.384-388 (2016).
2. Perez-Gussinye, M. & Reston, T. J. Rheological evolution during extension at nonvolcanic rifted margins: onset of serpentinization and development of detachments leading to continental breakup, J. Geophys. Res. 106,
3961-3975 (2001).
3. Reston, T. J. et al., Movement along a low-angle normal fault: the S reflector
west of Spain, Geochem. Geophys. Geosyst. 8, Q06002 (2007).
4. Christensen, N. I. Serpentinites, peridotites, and seismology, Int. Geol. Rev. 46,
795-816 (2004).
5. Coogan, L. A. et al. Chemical and thermal constraints on focussed fluid flow in
the lower oceanic crust, Am. J. Sci. 306, 389-427 (2006).
Comentários