Melt inclusions in phenocrysts reveals what is happening beneath the Mid-Ocean Ridge
In the mid-ocean ridge, pressure decrease induced by rising mantle convection produce magma, ascending and crystallization of it on the surface or beneath the Mid-Ocean Ridge form a oceanic crust. However, igneous process forming lower crust beneath is not well clarified. There are two end-member models that explaining crystallization and lower oceanic crust forming process. One end member model is gabbro glacier model that crystallization occurs on the base of the dike, cumulative downward and outward expansion forming oceanic crust (Morgan et al. 1993). The other is sheeted sill model that crystallization occurs over the wide range of depth from ascending magma, forming multiple sill beneath ridge axis (Figure1). Then these multiple sill formation is origin of the ridge axis (Kelemen et al. 1997).
Seismic reflection data can be used to evaluate magma crystallization model (Carbotte et al. 2013). Also, direct measurement of mid-ocean-ridge basalt (MORB) samples can allow us to investigate the mechanism of oceanic crust formation (Wanless et al. 2013, Saal et al, 2002). Magma erupted in the mid ocean ridge produced in the various depth below the basement of sea floor. Melt inclusions are trapped when phenocrysts crystallize at depth and they can tell us melt compositions and conditions during crystallization. Volatile (CO2 and H2O) contents in the melt inclusions can be used to calculate the crystallization depth because their solubility is directly proportional to the crystallization depth.
Wanless et al. 2013 show depth distribution of melt inclusion entrapment in the EPR (East Pacific Rise) and JFR (Juan de Fuca ridge) in the Pacific Ocean. Major crystallization depth induced by melt inclusion show “peaks” in 1~2.5km on the EPR and 2.5~4km in JFR, respectively from which we can deduce the presence of axial magma lenses beneath the ridge. However, crystallization depth is distributed widely below 9km for JdFR and 8.5km for EPR from ridge axis (Table 1). That might be interpreted as melt lens present deep along the crust. Crystallization depth distribution derived from phenocrysts melt inclusion entrapment should not be directly interpreted as the ratio of the crystallization depth. Because it is more difficult for phenocrysts crystallize in the deeper mantle.
Trace element compositions of the melt inclusions can be used to identify the magma evolution and differentiation. Those from EPR and JFR have La/Yb ratios between 0.7 and 1.4 and between 0.7 and 1.5 respectively (Wanless et al, 2013). It is close to N-MORB composition and relatively limited composition variations compared to those from MAR (Mid Atlantic Ridge) which has variation between 0.28 to 4.0 (Shimizu et al. 1996). In the JFR, Mg# (Ratio of MgO from MgO+FeO) show very limited value for each sampling site, valence and cleft phenocrysts sampling (Figure 2).
This result tells us that magma is homogenized on the melt lens located in the shallow crust. However, magma composition is already homogeneous below the melt lens and it may indicate the presence of deep melt pooling in the deep crust. In other words, magma is homogenized on the deep crust before ascending to shallow melt lens. Also, Crystallization depth distribution inferred from melt inclusion compositions in phenocryst has wide depth range. That’s why “sheeted sill” model (Kelemen et al, 1997) to describe lower crust formation is more reliable than other model. Furthermore, Wanless et al. (2013) and Carbotte et al. (2013) suggest the presence of deep magma pooling chambers in the crust-mantle boundary but there is no clear evidence (Figure3). Finding seismic evidence of magma pooling in crust-mantle boundary can be a further study.
Melt inclusions from the MAR show wide range of trace element compositions relative to JdFR and EPR. The difference in the composition of melt inclusions between EPR and MAR is attributed to different ridge spreading rate. The author interpret that magma homogenization at the deep crust is common in MOR, but degree of homogenization higher in fast spreading center. MAR is slow spreading center, and melt is not sufficiently homogenized in the lower part or crust and various composition of melt inclusion is erupted at the surface.

Figure 1 Crystallization depth of olivine phenocrysts
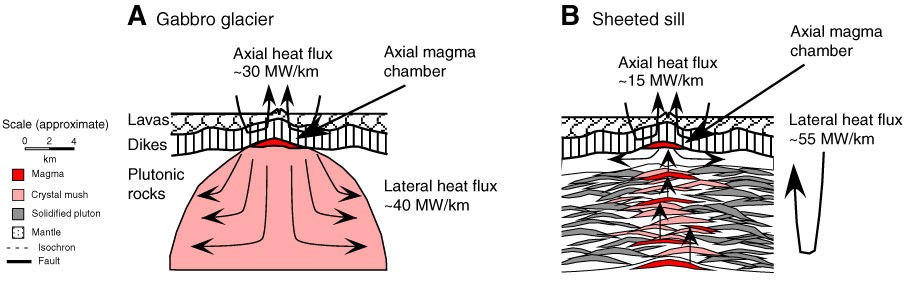
Figure 2 Two models for lower oceanic crust formation
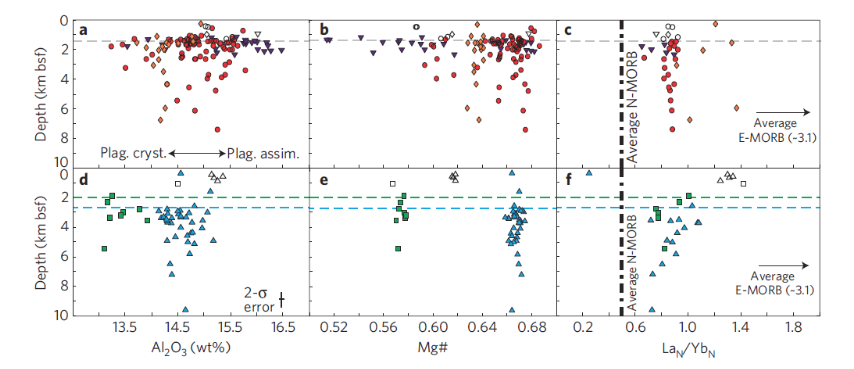
Figure 3 Compositional variations of melt inclusions against depth
Carbotte, S. M., Marjanović, M., Carton, H., Mutter, J. C., Canales, J. P., Nedimović, M. R., ... & Perfit, M. R. (2013). Fine-scale segmentation of the crustal magma reservoir beneath the East Pacific Rise. Nature Geoscience, 6(10), 866-870.
Kelemen, P. B., Koga, K., & Shimizu, N. (1997). Geochemistry of gabbro sills in the crust-mantle transition zone of the Oman ophiolite: Implications for the origin of the oceanic lower crust. Earth and Planetary Science Letters, 146(3), 475-488.
Nobumichi, S. (1998). The geochemistry of olivine-hosted melt inclusions in a FAMOUS basalt ALV519-4-1. Physics of the Earth and Planetary Interiors, 107(1), 183-201.
Morgan, J. P., & Ghen, Y. J. (1993). Dependence of ridge-axis morphology on magma supply and spreading rate. Nature, 364(6439), 706-708.
Saal, A. E., Hauri, E. H., Langmuir, C. H., & Perfit, M. R. (2002). Vapour undersaturation in primitive mid-ocean-ridge basalt and the volatile content of Earth's upper mantle. Nature, 419(6906), 451-455.
Wanless, V. D., & Shaw, A. M. (2012). Lower crustal crystallization and melt evolution at mid-ocean ridges. Nature Geoscience, 5(9), 651-655.